Rectivication in Schottky diodes in the infrared regime
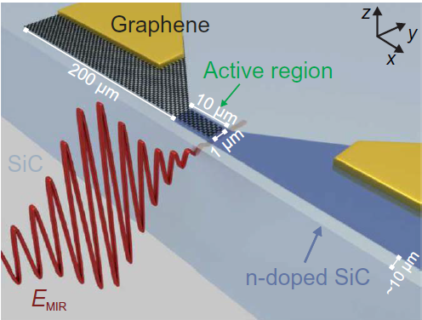
How fast does a diode actually work? If it is operated under ideal conditions, it can be recharged up to 2 trillion times per second – until now, it has not been possible to go faster in conventional high-frequency circuits. Using a physical trick, a research team from Friedrich-Alexander-Universität Erlangen-Nürnberg (FAU) and the University of Regensburg has managed to circumvent these externally imposed limitations and measure the inner workings of diodes at much higher frequencies. Their experiments thus extend theoretical predictions made by physicist Walter Schottky. They have published their results in the renowned journal Sciences Advances.*
A diode is probably the simplest active electronic component. It allows current to flow in only one direction and is therefore used for rectification. In the simplest case, all that is needed is a metal-semiconductor contact, also known as a Schottky diode after the physicist Walter Schottky, who was born in Berlin in 1886 and died in Pretzfeld near Erlangen in 1976. Its maximum speed is measured by how fast an applied voltage leads to a current flow. This is an extrinsic limitation that comes from the control, but not an intrinsic one – this could not be measured until now.
Schottky formulated a very successful model for the metal-semiconductor contact, i.e. the Schottky diode, in 1938 in the service of the Siemens company. It gets by with only a few assumptions and parameters and accurately describes the current-voltage curves in the DC case. It turned out that the equations also apply very accurately in the alternating current case, i.e. for signal transmission. The fastest Schottky diodes today can be operated under ideal conditions up to about two terahertz (THz), or 2 trillion oscillations per second. Recharging them faster is not possible with conventional high-frequency circuitry.
The goal of scientists from the Chair of Applied Physics at FAU and the Chair of Ultrafast Quantum Physics and Photonics at the University of Regensburg was now to measure diodes at even higher frequencies. This requires a trick: Instead of rapidly oscillating voltages, they used oscillating light fields in the mid-infrared range, which were irradiated onto the Schottky diodes in strong pulses. The electric field of the light was aligned to simulate the electric voltage on the diode without the need to bring electric charges to the diode. This was measured to see if the rectification of the diode resulted in a current, which could then be measured using the much slower electrical methods.
This unusual experiment places some demands on the diode: it must be able to withstand very strong light fields and be transparent. Therefore, epitaxial graphene was chosen as the metal and silicon carbide – a high-performance semiconductor with a long research tradition in Erlangen. In a high-temperature process, epitaxial graphene, a one-atom-thin layer of carbon that behaves metallically, can be grown onto the semiconductor. This smallest possible expansion is advantageous because then the electrons do not have to travel a long way through the metal. The FAU group led by Prof. Dr. Heiko B. Weber has already been able to show that this metal-semiconductor combination behaves like an ideal, transparent Schottky diode over a wide range of frequencies – from the DC voltage range up to 500 gigahertz.
To put Schottky physics to the test, the mid-infrared range is particularly exciting, because there the (electric) light fields can be chosen very high and – in contrast to visible light – no photoelectric effect takes place, which would complicate Schottky physics. Such methods have been worked out and carried out for years at the University of Regensburg in the research group of Prof. Dr. Rupert Huber.
The results were surprising at first: under the incidence of light, rectified currents appeared, but the rectification occurred in the opposite direction. To understand these results, one has to use Schottky’s model: One puts the experimentally determined electric field course of the light pulse into the Schottky formula and sees that with each light wave ultrafast current pulses are generated by electron tunneling, which already come very close to the observed values. However, this did not fit perfectly at first. But on closer inspection, this too can be understood: Those electrons that contribute to the current pulse just before the field reversal do not make it all the way through the diode and are thrown back again when the electric field reverses. Thus, they do not contribute to the current flow. The physicists combined the 1938 Schottky model with a correction they christened the “Dynamic Schottky Effect (DSE).” They then found an astonishing agreement: If the Schottky diode is measured under DC voltage and the rectified current at about 40 terahertz is calculated from this, the prediction is very accurate without any further adjustments.
The conclusion of the research team is thus: For the first time, it has been possible to operate an active electronic component with light fields. The 84-year-old Schottky model, which was designed for the DC case, continues to serve as a description when extended with the DSE correction presented here.
About the experiment
The work was carried out within the framework of the Erlangen Collaborative Research Center 953 “Synthetic Carbon Allotropes” and the Regensburg Collaborative Research Center 1277 “Emergent Relativistic Effects in Condensed Matter”.
* https://doi.org/10.1126/sciadv.abj5014